Coronal Mass Ejections: Understanding the Threat and Preparing for the Impact of a Carrington-Scale Event
- Louis
- May 2
- 9 min read
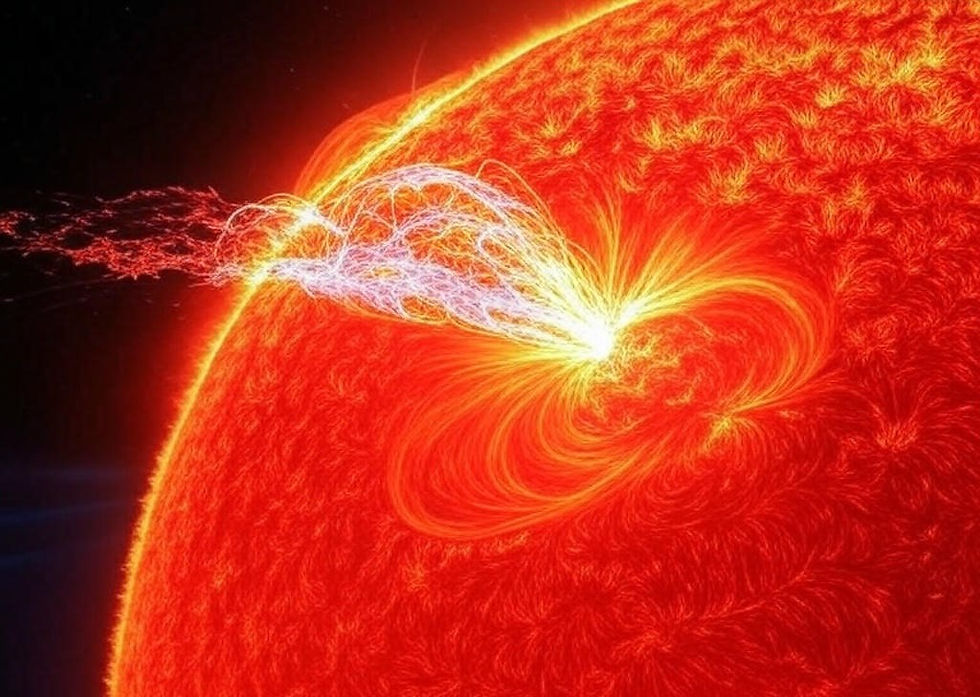
Coronal Mass Ejections (CMEs) are among the most powerful phenomena in our solar system, with the potential to cause widespread disruption on Earth. A Carrington Event-scale CME, named after the 1859 geomagnetic storm observed by British astronomer Richard Carrington, represents the benchmark for a catastrophic solar event. This article explores the nature of CMEs, the likelihood of a Carrington-scale event, the historical impacts of the 1859 storm, the potential modern-day consequences—including societal chaos—and how we can recognize and prepare for such an event.
What is a Coronal Mass Ejection (CME)?
A Coronal Mass Ejection (CME) is a massive burst of solar wind, plasma, and magnetic fields expelled from the Sun’s corona, often following a solar flare. These events release billions of tons of charged particles into space at speeds ranging from 250 to 3,000 km/s (Cliver & Svalgaard, 2004). When a CME impacts Earth, it interacts with the planet’s magnetosphere, potentially triggering a geomagnetic storm. The storm’s severity depends on the CME’s speed, magnetic field orientation, and energy. The Carrington Event of 1859 involved a CME with an energy release of approximately 10^32 ergs and a velocity of around 2,300 km/s, making it one of the most powerful on record (Tsurutani et al., 2003). This level of intensity can cause global disruption, as evidenced by historical and modern analyses.
Probability of a Carrington Event-Scale CME
CMEs occur frequently during the Sun’s 11-year solar cycle, with larger events more likely during solar maximum. The peak of Solar Cycle 25 is expected in mid-2025 (NOAA Space Weather Prediction Center, 2023). While most CMEs are minor, a Carrington-scale event is rare but plausible:
Historical Frequency: Ice core data, which record nitrate spikes from past solar storms, suggest a Carrington-scale event occurs approximately once every 150 years (McCracken et al., 2001). The last such event was in 1859, meaning we are within the statistical window for another occurrence.
Modern Estimates: A 2012 study in Space Weather estimated a 12% chance of a Carrington-scale event per decade (Riley, 2012). NASA concurs, noting an increased likelihood during solar maximum (NASA, 2023).
Near Misses: In July 2012, a Carrington-scale CME narrowly missed Earth, passing through our orbit just nine days after Earth’s position. Had it struck, the global damage and disruption would have been catastrophic.
Historical Impacts of the Carrington Event
The Carrington Event of 1859 provides a historical baseline for understanding the effects of a massive CME:
Auroras: The geomagnetic storm produced auroras visible at unusually low latitudes, including the Caribbean (geomagnetic latitude ~20°N). Eyewitness accounts describe vibrant green, red, and purple displays bright enough to read newspapers by, extending to Australia as far north as Brisbane (geomagnetic latitude ~35°S) (Loomis, 1860; Neumayer, 1860).
Telegraph Disruptions: The storm induced geomagnetically induced currents (GICs) in telegraph lines, the primary electrical infrastructure of the time. Operators reported sparks, fires, and some systems operating without power due to induced currents. In the U.S., telegraph communication between Boston and Portland was disrupted for hours (Boteler, 2006).
Duration: The storm’s effects peaked within the first 24–48 hours but persisted for several days, with auroras and magnetic disturbances recorded globally (Cliver & Svalgaard, 2004).
Potential Impacts of a Carrington Event Today
Modern society’s reliance on technology amplifies the risks of a Carrington-scale CME. The impacts would span multiple sectors, with cascading effects leading to societal chaos.
Power Grid Failures
Mechanism: GICs from a geomagnetic storm can overload power transformers, causing widespread blackouts. The 1989 geomagnetic storm, only one-fifth the strength of the Carrington Event, caused a 9-hour blackout in Quebec by damaging transformers in Hydro-Québec’s grid (Bolduc, 2002).
Scale: A Carrington-scale event could cause outages across entire continents, lasting weeks to months. Lloyd’s of London (2013) estimates global damages at $2.6 trillion, with transformer replacement delayed by supply chain constraints, as global manufacturing capacity for large transformers is limited.
Impact: Loss of power would disrupt water treatment, refrigeration, and healthcare systems. In Australia, where 30% of food is imported (Australian Bureau of Statistics, 2023), blackouts could halt supply chains, leading to shortages within days, as demonstrated by the 2022 Optus outage, which disrupted logistics for several days (Australian Cyber Security Centre, 2022).
Satellite and Communication Disruptions
Mechanism: CMEs emit charged particles that can damage satellite electronics and disrupt radio signals. The 2003 Halloween storms, less intense than the Carrington Event, damaged 10% of the global satellite fleet, costing $640 million (NOAA, 2004).
Scale: A Carrington-scale event could disable GPS, satellite phones, and internet services like Starlink, which are critical for rural areas in Australia (Australian Government, 2023). The 1989 storm caused radio blackouts affecting aviation in Australia (IPS Radio and Space Services, 1989).
Impact: Loss of communication would isolate communities, hinder emergency responses, and disrupt financial systems reliant on GPS timing, such as banking transactions, which require precise synchronization (National Academy of Sciences, 2008).
Transportation and Aviation
Mechanism: Geomagnetic storms disrupt high-frequency (HF) radio communications and GPS, essential for aviation, while also increasing radiation risks at high altitudes. The 2003 Halloween storms forced airlines to reroute polar flights to avoid radiation exposure (NOAA, 2004).
Scale: A Carrington-scale event could ground flights globally, as airlines lack contingency plans for complete GPS loss (International Air Transport Association, 2023).
Impact: Disrupted aviation would halt the transport of perishable goods, such as 40% of Australia’s seafood imports (Department of Agriculture, Fisheries and Forestry, 2023), exacerbating food shortages and economic strain.
Societal Chaos from Communication and Supply Chain Disruptions
The loss of power and communication would trigger cascading societal effects, leading to chaos and potential lawlessness:
Communication Breakdown: Without satellite and radio communication, emergency services would struggle to coordinate responses, as seen during the 2011 Queensland floods, where isolated communities waited over a week for aid (Queensland Government, 2011). Rural Australia, home to 28% of the population (Australian Bureau of Statistics, 2023), would be particularly vulnerable, unable to receive updates or call for help.
Supply Chain Collapse: Blackouts would halt food and fuel distribution. Australia’s reliance on imports and long supply chains means supermarkets could empty within days, as occurred during the 2020 COVID-19 panic buying, where shelves were stripped in hours (Australian Bureau of Statistics, 2020). The 2021 Colonial Pipeline ransomware attack in the U.S., which caused fuel shortages across the East Coast, illustrates how quickly panic can escalate (U.S. Department of Energy, 2021).
Lawlessness and Looting: Historical crises demonstrate that resource scarcity can lead to civil unrest. During the 2011 Christchurch earthquake, looting increased as power outages and isolation fuelled desperation (New Zealand Police, 2011). Similarly, the 2003 Northeast U.S. blackout saw a spike in crime (NYPD, 2003). In a Carrington-scale event, urban centres like Sydney or Melbourne could experience looting and violence as food, water, and fuel become scarce, with police response hampered by communication failures.
Advance Notice of a CME
Detection: Space weather monitoring systems, such as NASA’s Solar and Heliospheric Observatory (SOHO) and NOAA’s Deep Space Climate Observatory (DSCOVR), detect CMEs shortly after they erupt. Solar flares are observed in real-time via X-ray and optical telescopes, while CMEs are tracked using coronagraphs (NOAA Space Weather Prediction Center, 2023).
Warning Time: A Carrington-scale CME, traveling at 2,300 km/s, would reach Earth in 17–24 hours, as observed in 1859 (Cliver & Dietrich, 2013). Slower CMEs might take 2–3 days. DSCOVR, positioned at the L1 Lagrange point 1.5 million km from Earth, provides a final warning 15–60 minutes before impact (NOAA, 2023).
Challenges: Not all CMEs are Earth-directed, and predicting their magnetic orientation—a key factor in storm severity—is challenging. The 2012 near-miss CME was only identified as Carrington-scale after post-event analysis (Baker et al., 2013).
Recognizing a CME Impact from the Ground
If advance notice is missed, a Carrington-scale CME’s impact would be unmistakable:
Auroras: Brilliant auroras would appear at low latitudes, visible across Australia, even in Darwin (geomagnetic latitude ~22°S). Colours would include green, red, and purple, lasting days, as predicted by geomagnetic models (Pulkkinen et al., 2012).
Power and Communication Failures: Sudden blackouts, GPS failures, and radio blackouts would occur simultaneously. Transformers might spark or fail, as seen in the 1989 Quebec event, where Hydro-Québec’s grid collapsed (Bolduc, 2002).
Environmental Signs: Increased radiation levels could trigger alerts on Geiger counters, and aviation disruptions would be reported if some communication channels remain functional (NOAA, 2004).
Preparation for a Carrington Event-Scale CME
Government Preparation
Grid Hardening: Governments can install GIC-blocking devices on transformers, as recommended by the U.S. Federal Energy Regulatory Commission (FERC, 2016). In Australia, the Australian Energy Market Operator (AEMO) could prioritize protecting long transmission lines, such as the 1,000 km Basslink interconnector (AEMO, 2023).
Satellite Protection: Satellites can be placed in safe mode during solar storms, as was done during the 2003 Halloween storms (NOAA, 2004). Australia’s space agency could coordinate with international partners to ensure redundancy in communication systems.
Emergency Plans: Stockpiling transformers and developing blackout response plans, as suggested by the National Academy of Sciences (2008), would reduce recovery time. Public education campaigns, such as those outlined in Australia’s 2023 National Risk Assessment, can prepare citizens for prolonged disruptions.
Individual Preparation
Bunkers and Supplies: A private bunker stocked with food, water, and medical supplies for at least a month can mitigate shortages and can protect against lawlessness. Stockpiling essentials ensures survival during supply chain disruptions.
Protecting Back-Up Power Systems:
Solar Panels and Generators: Solar panels or portable generators can provide off-grid power, as recommended by the Australian Red Cross (2023). However, their electronic components, such as charge controllers and inverters, are vulnerable to the induced currents from a CME.
Faraday Cages for Protection: A Faraday cage is a conductive enclosure that blocks electromagnetic fields, protecting electronics from a CME’s effects (U.S. EMP Commission, 2008). Commercially developed and tested Faraday cages are advised, but functional Faraday cages can also be constructed at home from readily available materials. Detailed instructions on Faraday cage construction is beyond the scope of this article, but the basic steps are outlined below:
Materials: Use a metal container, such as a steel ammo can or aluminium toolbox, with a tight-fitting lid to ensure no gaps. Even a microwave oven (unplugged) can work, as its design already blocks electromagnetic waves.
Insulation: Line the inside with a non-conductive material, such as cardboard, foam, or rubber, to prevent electronics from touching the metal, which could conduct residual currents.
Sealing: Seal the lid with conductive tape, such as copper or aluminium tape, to close any gaps where electromagnetic waves could penetrate. Ensure all seams are covered to maintain the cage’s integrity.
Grounding (Optional): While not always necessary for small setups, grounding the cage by attaching a wire from the container to a grounding rod driven into the earth can enhance protection, especially for larger systems (FEMA, 2010).
Storage: Place critical power components—such as solar charge controllers, inverters, battery management systems, or generator circuit boards—inside the cage. Keep the cage closed during the CME event to ensure protection.
Practical Example: A solar panel system with a charge controller can be stored in a Faraday cage made from a metal tin, insulated with foam, and sealed with copper tape. Post-CME, the system can be used to charge batteries or power essential devices like lights or a small refrigerator.
Protecting Communication Systems:
Hand-Crank Radios: These provide access to emergency broadcasts without relying on external power, as recommended by the Australian Red Cross (2023). Their circuitry must be protected to remain functional after a CME.
Faraday Bags for Small Devices: For portable radios, satellite phones, or walkie-talkies, use a Faraday bag—a portable, conductive pouch made of metalized fabric. These are commercially available, but a DIY version can be made by wrapping devices in at least three layers of aluminum foil and sealing them in a ziplock bag to prevent tearing (FEMA, 2010). Each layer of foil should be separated by a thin insulator, such as plastic wrap, to ensure no gaps.
Construction Tip: Ensure the foil completely envelops the device with no exposed areas. Test the bag by placing a mobile phone inside and calling it—if it doesn’t ring, the bag is blocking signals effectively (U.S. EMP Commission, 2008).
Practical Example: A hand-crank radio and a set of walkie-talkies can be stored in a DIY Faraday bag made by wrapping the devices in three layers of aluminum foil, separated by plastic wrap, and sealed in a ziplock bag. This ensures they remain operational for post-CME communication, allowing access to emergency broadcasts or local coordination.
Additional Devices: Protect other communication tools, such as ham radios or spare smartphone batteries, in similar Faraday enclosures. Ham radios, in particular, can be a lifeline for long-range communication when satellites fail, as noted in disaster preparedness guides (Australian Government, 2023).
Self-Sufficiency: Stockpiling seeds and water purification systems supports long-term survival, especially in rural Australia, where isolation is a risk (Australian Government, 2023). Paper maps and a compass ensure navigation without GPS, which is critical given the expected disruption to satellite systems.
Conclusion
A Carrington Event-scale CME, with a 12% chance per decade of occurrence, poses a significant threat to modern society (Riley, 2012). The 1859 event demonstrated its global reach, and today, it could cause trillions in damages, widespread blackouts, and societal chaos, as evidenced by historical crises like the 2011 Christchurch earthquake (New Zealand Police, 2011). With 17–24 hours of warning, governments and individuals can prepare by hardening infrastructure, stockpiling supplies, and protecting critical systems. Civilians can safeguard back-up power and communication systems using Faraday cages and bags, ensuring functionality post-CME. Recognizing the signs—auroras, power failures, and communication disruptions—enables a swift response if notice is missed. As we approach the 2025 solar maximum, proactive preparation is essential to mitigate the impacts of this low-probability, high-impact event.
Comments